We need to talk about Circularity in Renewables
- Vasilakis Nikos
- 15 Ιουν 2023
- διαβάστηκε 7 λεπτά
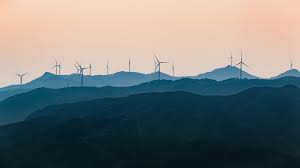
The renewable energy sector promises to tap into limitless sources of energy while tackling pollution and climate change.
However, the materials needed to capture and store this energy are finite. As the industry scales at pace, renewable infrastructure designed within a ‘take-make-waste’ linear system could contribute to greenhouse gas emissions and biodiversity loss. To prevent this solution becoming a problem, renewable energy infrastructure needs to be built for a circular economy.
It is unquestionable that renewable energy needs to be part of the solution to climate change. With many countries committing at COP26 to reach net-zero carbon emissions by 2050, renewable energy infrastructure is set to scale rapidly.
New onshore wind power capacity added in 2020 was twice that installed in 2019 and the US, Europe, China, and other countries have laid out plans to ramp up investments in renewables. These have been further accelerated by Russia’s recent invasion of Ukraine, with European Commission President Ursula von der Leyen stating, “The quicker we switch to renewables and hydrogen, combined with more energy efficiency, the quicker we will be truly independent and master our energy system.”
However, while vital to reach a net-zero ambition, the renewable energy industry is not without its challenges. In early 2020, images of diggers burying rows of decommissioned wind turbine blades against a picturesque mountain range in Casper, Wyoming, captured headlines. The coverage gave credence to renewable energy sceptics who question the environmental credentials of current renewable technologies, with detractors labelling the turbines "Not so green energy”.
While these critics are not without their own agendas, it is certainly true that as the wind industry grows this problem is set to extend – it is estimated there will be 43 million tonnes of wind turbine blade waste by 2050 – and the blades landfilled in Wyoming will not be the only example of waste from the renewable energy sector entering the environment. Lithium-ion battery waste is predicted to reach 2 million tonnes annually by 2030 and the International Renewable Energy Agency (IRENA) estimates that there will be up to 78 million tonnes of solar panel waste by 2050.

This waste is currently an inevitability, the result of design and materials choices centred around two key factors: energy output and cost. These design choices have enabled renewables to compete with an established fossil-fuel industry.
The need to integrate a circular economy approachinto the renewable energy transition is clear.
While there are barriers in the current system to overcome, a number of pioneering businesses and policymakers are forging ahead to create solutions. Their efforts, especially if bolstered by others, can lead to a renewable energy sector that not only produces cleaner energy but is delivered via infrastructure that, by design, eliminates waste and pollution, keeps the materials it needs in use, and regenerates nature. This model, a circular economy, will lead to greater benefits for the environment, people, and business.
It's time for a redesign. Solar panels and wind turbine blades ending up in landfill are flaws in product and business model design. In the same way, allowing materials to become waste after use while virgin materials are extracted to make new renewable energy technologies is a system design flaw.
Adopting a circular economy approach to the development of the renewable energy sector offers solutions to these shortcomings. Indeed, interest in how a circular economy approach can help tackle the 45% of greenhouse gas (GHG) emissions from how we make products and manage the land is beginning to take its place as part of the global climate agenda. It is central to the vision of the US Department for Energy’s National Renewable Energy Laboratory, and has been highlighted as underpinning “the “sustainability credentials” of Europe’s renewable energy transition by the European Environment Agency (EEA). It is also prominent in China’s 14th Five-Year Plan in relation to batteries as well as the metals needed for solar panels and wind turbines.
Integrating a circular economy approach into renewable energy infrastructure development – securing resources and tackling materials-related environmental impacts – requires a redesign of technologies, business models, and policies. It offers the opportunity to create a better system, in which waste and pollution are eliminated, material consumption is reduced, products and materials are circulated, and space is left for nature to thrive.
So where to begin?
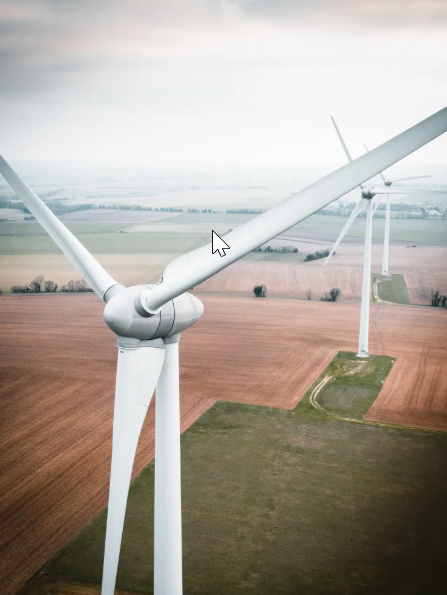
One clear circular economy approach is to extend the lifetimes of existing technologies and in turn significantly reduce material needs. As a general rule, the expected lifetime of both wind and solar technology is around 25 years. Extending the life of a 500 megawatt wind farm by 10 years could over the course of a century reduce demand for copper alone by around 4,400 tonnes, while reducing the greenhouse gas (GHG) emissions and impacts on biodiversity associated with copper mining and production.
Perhaps the simplest example of lifetime extension is regular maintenance. In wind farms, keeping blades and components clean and in good working order reduces downtime and costs. Service concepts have become increasingly sophisticated as technology has developed, with providers and consortiums, such as Siemens and BladeSave, offering condition monitoring service systems which use a series of sensors to detect anomalies, vibrations, and weather conditions, and advise corrective actions early.
In the solar sector, an economic model has been proposed that focuses on maintenance at regular intervals. If applied, the model predicts that modules installed today should be viable for 50 years before they need to be replaced.
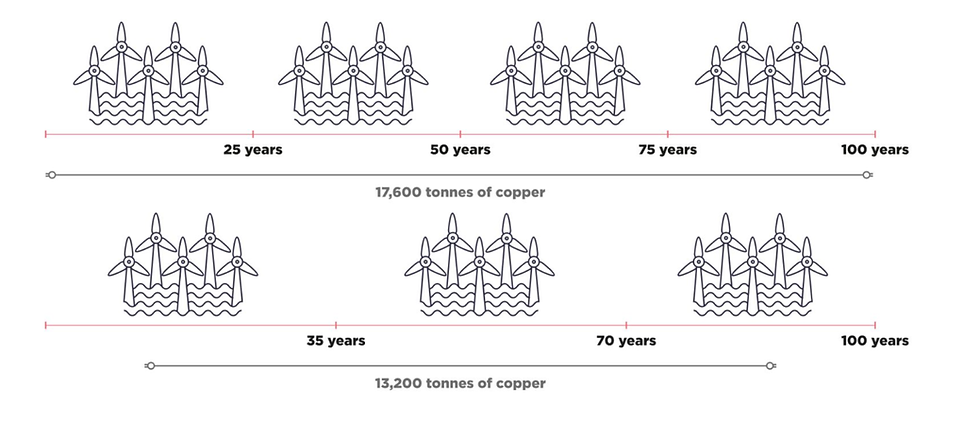
Repairing and updating equipment is also impactful. The fastest moving parts in a turbine are subject to the greatest wear and tear, namely the blades and the gearbox. Leading edge erosion is common in turbine blades, for example, but polymer pastes, vacuum assisted crack injections, and patch repairs have been developed to mend – in situ – cracks, pitting, delamination, and other problems. Retrofitting individual blade controls can minimise the strain on them, making the parts last longer.
When they do eventually wear out, many parts can be remanufactured to prolong the overall life of the asset. Components such as gearboxes, generators, bearings, and rotors that are predominantly made of steel can be readily remanufactured to return them to their original specification. Remanufacturing is an ‘inner loop’ of the circular economy as it maintains more of the embedded energy and labour that went into making the original components compared to ‘outer loop’ strategies like recycling.
In a lifetime extension scenario, worn components are replaced while keeping the towers, foundations, and electrical grid installation in place. Installing refurbished blades and nacelles in five offshore wind turbines at Bockstigen in Sweden after 20 years in service increased their capacity from 2.75 megawatts to 3.3 megawatts and extended their productivity by 15 years.
The opportunities of maintaining and refurbishing renewable energy assets are increasingly being taken up by pioneering organisations.
Upstream design strategies are crucial to long-term material availability. While lifetime extension keeps materials in use and lessens environmental impacts, leaving space for nature to thrive, in certain circumstances there is a strong argument for full repowering – entirely replacing the technology.
No matter the renewable energy technology in question, the solution is to get the use cycles right. Wind farms that exist today, if they are effective, need to be maintained, refurbished, and repaired in order to maximise environmental and other benefits. But if a wind farm could be more effective were it repowered then there need to be considerations around where the materials to build the new infrastructure come from and where materials from the old infrastructure go so that they keep adding value in the economy.
That requires technology providers to source materials from within the industry and the economy more broadly, rather than turning to extraction of finite materials. It requires decommissioning guidance and financing to be made available to ensure products and components are reused or, if that isn’t possible, their materials recycled (either within the renewable energy industry or in other industries), and designing for these purposes. Policy is needed to support reuse when it is appropriate and, when it isn’t, to support systems that keep the materials in the economy and out of landfill.
Crucially, all possible use cycles should be planned for at the design stage. As Radjou and Prabhu in their book Frugal Innovation argue, “over 70% of a product’s life-cycle costs and environmental footprint is determined during its design phase,” and once decisions are implemented, it’s hard to reverse their impacts.
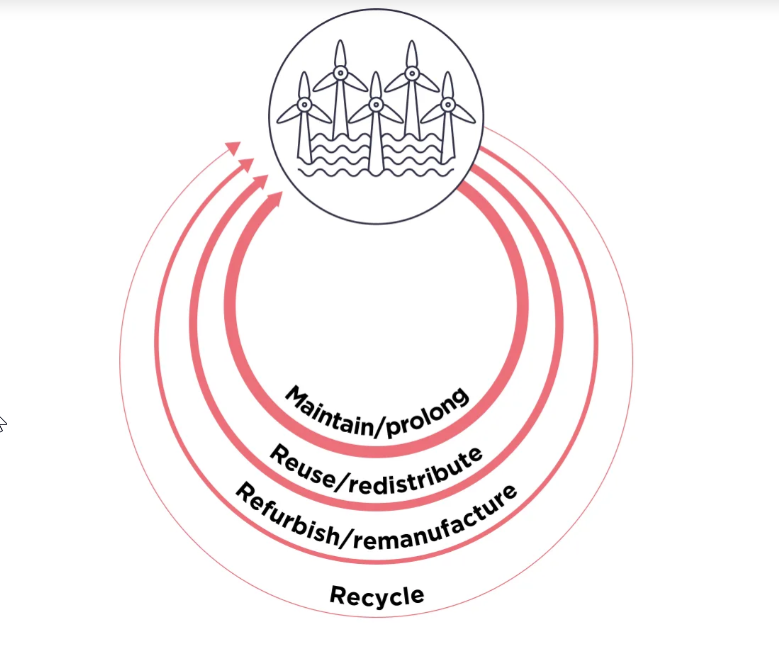
Design for reuse.
Among the strategies recommended for PV panel design by the International Energy Agency (IEA) are: minimising the use of hazardous and hard-to-recover materials, particularly in the metallic backsheet; and choosing reversible adhesive bonds, encapsulants and sealants to aid disassembly. Industry collaboration to standardise panel design is another important strand of circular design, alongside the introduction of material passports to track and disclose material origin and composition. The EPEAT ecolabel includes criterion on material recovery targets and requires material composition datasheets to be made available to recyclers.
At the moment, only a handful of companies consider end-of-use at the design stage.
While caution should be exercised, however, shifting the focus upstream, to the design stage, could be transformative for the renewable energy sector.

Policymakers can play a key role in enabling the economics to work. For example, the European Union (EU) Waste Electrical and Electronic Equipment (WEEE) directive mandates that the cost of collection, sorting, and reuse or recycling of technologies, which includes solar panels, be borne by ‘producers’.
The European Committee for Electrotechnical Standardisation (CENELEC) has developed a supplementary standard, specific to PV panel collection and treatment, to assist recyclers with recovery of the semiconductor materials. But, as this standard is voluntary there is still room for much-needed development in this area.
Beyond collection and recycling, policy development is also needed to support further adoption of reuse opportunities. Looking to the EU again, the region’s Waste Framework Directive currently defines reuse of an item as “any operation by which products or components that are not waste are used again for the same purpose for which they were conceived.” This is problematic if technologies move between different end uses: it is already preventing reuse of EV batteries for utilities and off-grid storage as this is considered downcycling and not permitted.
Source:MacArthour Foundation
Comments